In situ vaccination with oncolytic viruses
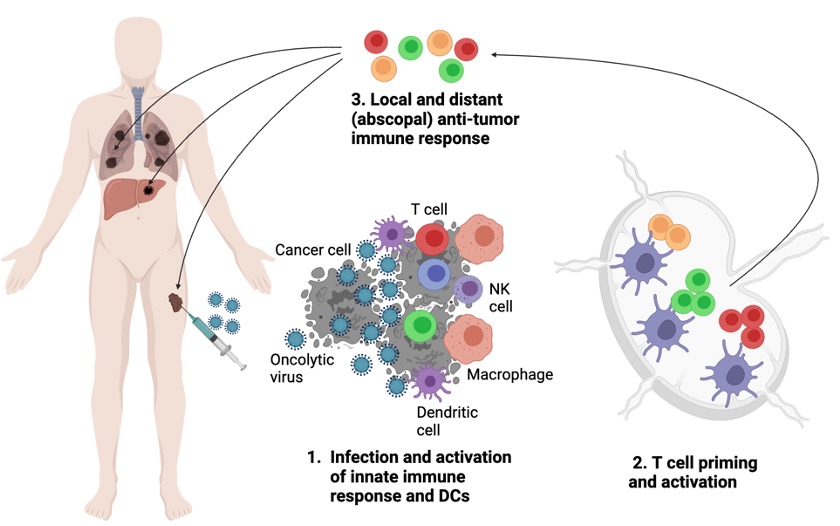
Figure 1. Oncolytic viruses promote local and abscopal anti-tumor immune response
Oncolytic viruses are an emerging class of cancer therapeutics based on the viruses that have specific predilection to infect and replicate in cancer cells while generally sparing immune cells. In addition to directly inducing cancer cell lysis, oncolytic viruses serve as strong activators of both innate and adaptive immune response. This leads to activation of antigen-presenting cells such as dendritic cells and priming of T cells that are specific not just for viral, but also for tumor antigens. To this effect, oncolytic viruses serve as in situ vaccines, leading to generation of systemic anti-tumor T cell response active both at the virus-injected (local) and distant (abscopal) sites.
As a model oncolytic virus, we study Newcastle Disease Virus (NDV), an avian paramyxovirus that carries a strong predilection for replication in human cancer cells. A major focus of our laboratory is focused on the understanding of the mechanistic basis underpinning the anti-tumor and anti-viral immunity generated by oncolytic viruses and on the development of novel therapeutic strategies based on engineered oncolytic viruses and combinations.
Evolution of anti-viral and anti-tumor immunity in response to oncolytic viral therapy
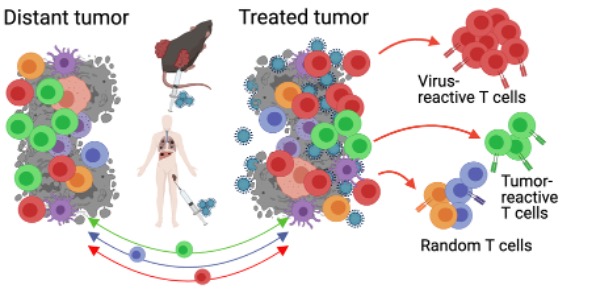
Figure 2. Balance of anti-viral and anti-tumor T cells generated by oncolytic viral therapy and its impact on anti-tumor immunity is not well established
Studies of oncolytic viruses to date have primarily used increase in tumor-infiltrating lymphocytes (TILs) as a readout of immune activation, commonly referred to as “converting cold tumors into hot”. However, while this biomarker may be informative with other immunotherapies such as immune checkpoint blockade, in the context of oncolytic virus therapy such readouts are highly non-specific. It is unknown whether the increase in TILs with OV therapy represents predominantly tumor-reactive, OV-reactive, or bystander T cells. Second, while a strong anti-OV response T cell response is likely generated with OV therapy, it is unknown whether these OV-reactive T cells positively or negatively impact anti-tumor immunity. Lastly, it is unknown how different aspects of oncolytic virus biology, including replication capacity, expression of therapeutic transgenes, and combination therapies impact the balance between the antiviral and anti-tumor immunity. We are actively pursuing these questions in the lab.

Figure 3. Intratumoral oncolytic virus therapy normalizes the TCR repertoire across the tumor sites. Top: Overall repertoire breadth and distribution of shared TCR clones across the treated and distant tumors. Bottom: unbiased identification of virus- vs. tumor-reactive TCRs demonstrates their dominance, phenotypic states, and distribution across the treated and distant tumors.
Potentiation of T cell-based immunotherapies by oncolytic viruses

Several features of oncolytic virus therapy generate a strong rationale for their combinations with other agents. For example, modification of the tumor microenvironment by the viruses improves sensitivity of the tumor to cytotoxic agents such as chemotherapy and targeted agents. Priming of tumor-specific immune responses generates a pool of T cells the efficacy of which is further potentiated by systemic immunomodulatory antibodies such as immune checkpoint inhibitors. Finally, by modification of the tumor microenvironment and improved priming, oncolytic viruses can increase the efficacy of adoptive T cell therapy. These features, combined with potential for engineered oncolytic viruses create opportunities for studies of biology and therapeutic efficacy of different combinations.

Figure 4. NDV increases tumor lymphocyte infiltration and promotes response to systemic immune checkpoint blockade (ICB). A. Treatment schema (B16 model). B. Numbers of intratumoral CD8+, CD4+FoxP3- and CD4+FoxP3+ T cells in distant tumors calculated from flow cytometry. C. Survival with NDV+anti-PD-1 or anti-PD-L1 combinations.
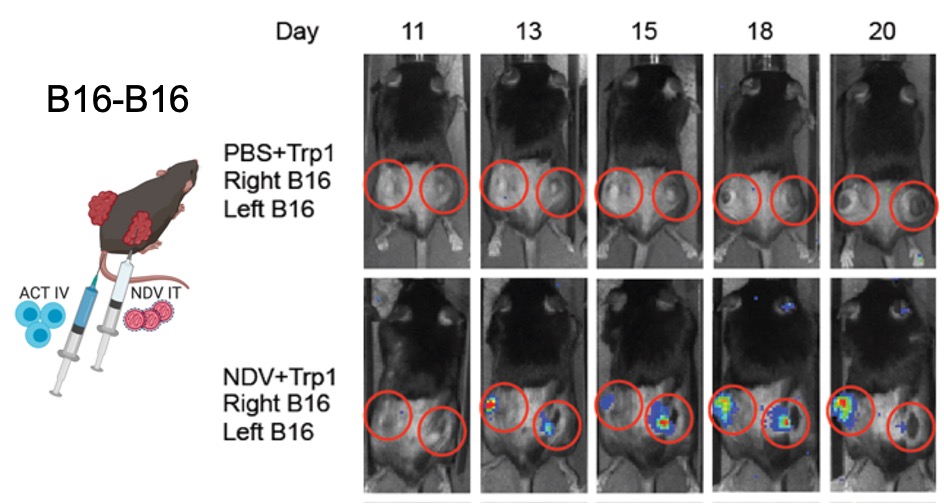
Figure 5. NDV induces tumor infiltration with tumor-specific adoptively-transferred T cells. Left: Treatment schema utilizing intratumoral NDV in combination with systemic ACT of luciferase-expressing transgenic T cells targeting B16 Trp1 antigen. Animals were implanted with bilateral flank B16 tumors; only right tumor was injected with NDV. Right: Infiltration of Trp1 lymphocytes into the NDV-treated and distant tumors.
Engineering novel oncolytic viruses as cancer immunotherapeutics
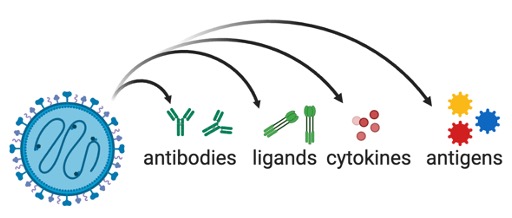
Figure 6. Oncolytic viruses can be engineered to deliver therapeutic payloads to tumors.
In addition to directly activating the innate and adaptive immune response, oncolytic viruses can be used as delivery vehicles for therapeutic transgenes directly into the tumor. Depending on the application, such transgenes can range from strategies to boost the innate or adaptive immune cells (e.g. through expression of cytokines) to expression of tumor antigens to stimulate tumor-specific immunity in a vaccine-like fashion. We have generated a number of engineered NDVs expressing different immunostimulatory transgenes and demonstrated that this can significantly improve the therapeutic potential of these agents.

Figure 7. Engineering strategies can improve therapeutic efficacy of oncolytic virus. Left: intratumoral therapy with wild-type NDV identifies inducible costimulator (ICOS) as one of the highest upregulated genes in tumor. Right: therapeutic effect of NDV encoding ICOS ligand (ICOSL) in bilateral flank melanoma mouse models.
Mechanisms of response and resistance to immunotherapy in gynecologic cancers
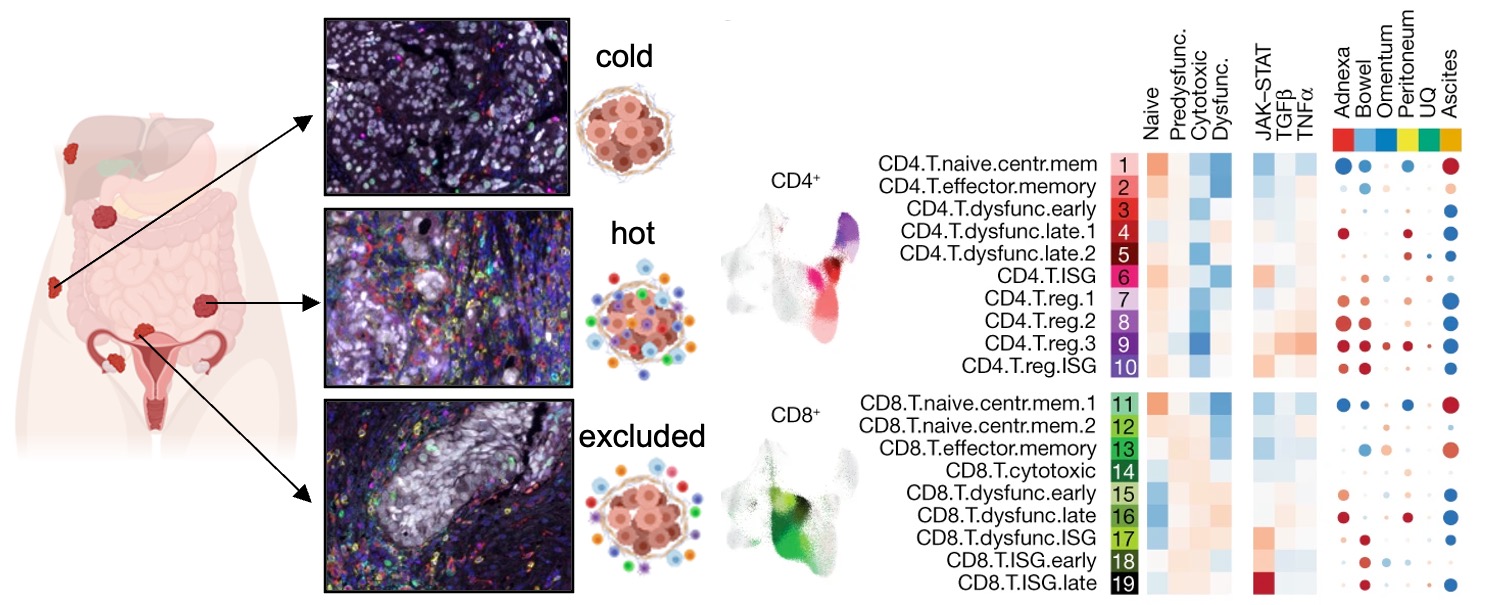
Figure 8. Ovarian tumors from the same patients exhibit substantial inter-tumor immune heterogeneity. Left: multiplex IF images of several tumor sites isolated from the same patient. Right: multi-site single-cell RNA seq analysis demonstrating differences in predominant T cell phenotypes across the sites.
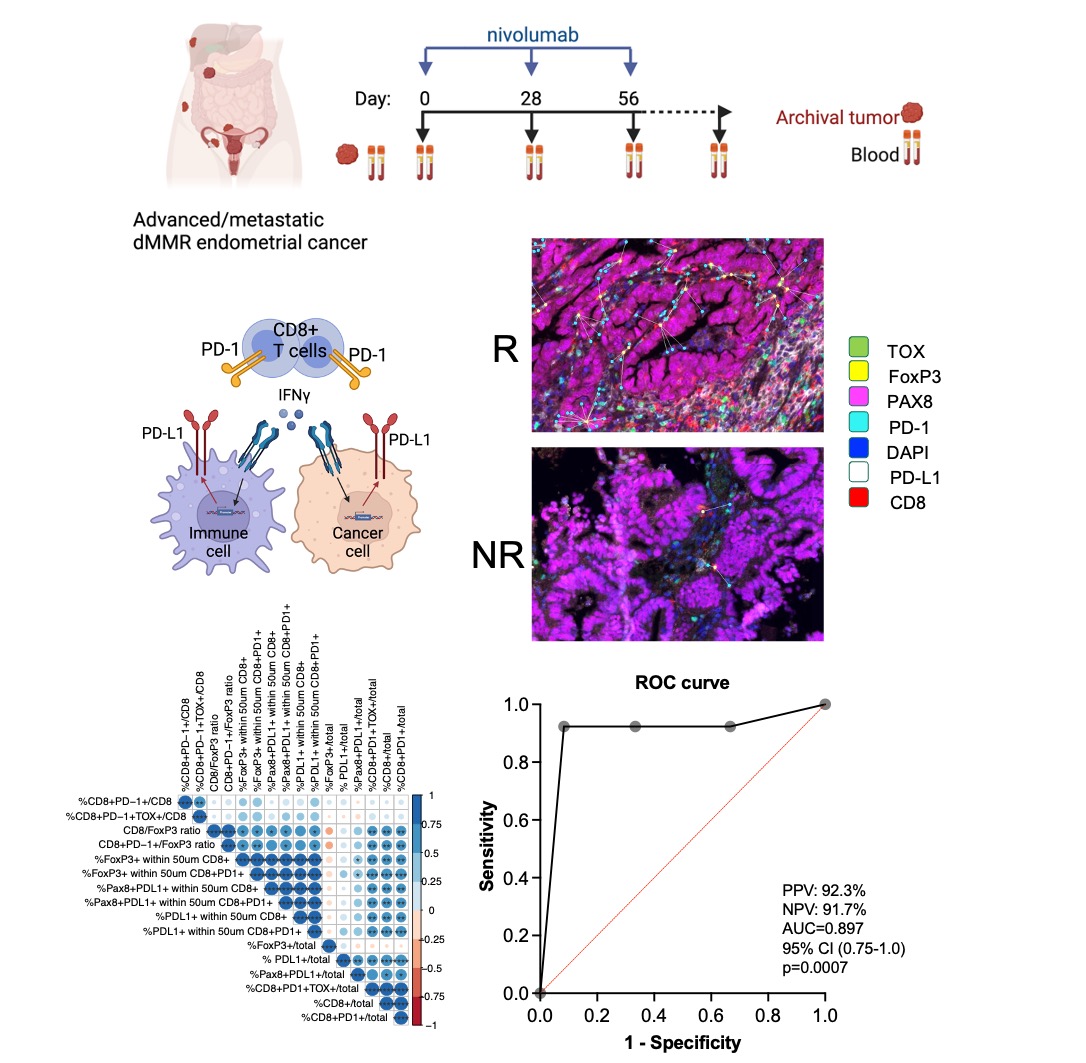
Figure 9. Tumor microenvironment parameters predict therapeutic response in dMMR endometrial cancer.
Several ongoing projects include:
- Evolution of immune response to intraperitoneal oncolytic virus therapy in patients with advanced ovarian and colorectal cancers
- Understanding of response to differential sequencing of immunotherapy and chemoradiation in locally advanced cervical cancer
- Predictors of response to immune checkpoint blockade in patients with endometrial and ovarian cancers
Understanding tumor cell-intrinsic mechanisms that drive immune recognition or resistance

Figure 10. Various tumor cell-intrinsic mechanisms ultimately control their recognition by the immune system
There is a growing appreciation that tumor genomic features shape the phenotypes of the tumor microenvironment, including the recruitment, infiltration, and functional states of T cells as well as responsiveness to immunotherapy. Such features include genomic alterations that lead to generation of immunogenic neoantigens that promote immune recognition (e.g. tumor mutation burden), but also alterations in antigen presentation machinery (e.g. HLA loss) that inhibit the immune response. Tumor genomic alterations in addition drive activation of tumor cell-intrinsic signaling pathways that can promote (e.g. type I IFN) or inhibit (e.g. beta-catenin) immune response. Understanding how cancer genetics defines tumor cell-intrinsic immune signaling as well as recognition by the innate and adaptive immune cells is critically important for the design of effective immunotherapeutic interventions. By using genetically-engineered syngeneic tumor models and organoids, we are evaluating how specific genetic alterations relevant to human cancers drive immune recognition.
